“The Universe has spoken and we have understood.” — David Blair, an astrophysicist at the University of Western Australia and LIGO collaboration memberd businessman
Researchers from the Indian Institute of Technology Gandhinagar, in collaboration with the Chennai Mathematical Institute, have made a major contribution in deciphering the fainter components of gravitational-wave signals (named as GW190412) that arose from the amalgamation of two asymmetric black-holes. Soumen Roy (a Ph.D. student) who is working with Dr. Anand Sengupta (faculty in Physics at IITGN), developed a novel technique (jointly with Dr. KG Arun from CMI) to pull out the feeble yet beautiful cosmic signals from the gravitational-wave emissions. It led him to practically ‘hear’ the musical ‘hummm’ that had only existed in theory before this study. For any passionate researcher, it is like a fairytale becoming a reality!
What are Gravitational Waves?
To understand these waves better we need to think about how interactions happen. If we look at the universe, it is basically made up of fundamental particles that interact with one another and these associations give rise to everything we observe around us. For example, two people are able to see each other because of photons (basic unit of light) interacting at different levels.
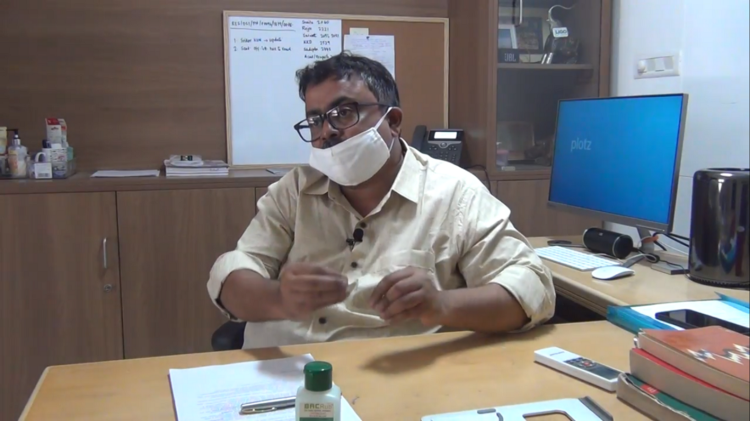
Now, we need a stage for this process to work and these interactions to take place. This is what we call space-time and it determines the entire geometry of the universe. If we consider this stage as a pond of water and disturb it at some point, this perturbation will eventually travel out through the entire pond.
“The exact same thing happens with space-time. A disturbance in any part of it (rotating blackholes or spinning stars or simply a person waving their hands) travels out to everywhere in the universe in the form of ripples. In simple terms, gravitational waves can be thought of as a category of these ripples in this space-time background,” explained Dr. Sengupta.
How are these Waves Detected?
The best way to detect anything is by analyzing how it interacts with matter (any physical substance that occupies space and possesses mass). Gravitational waves interact very feebly with the matter. Say, we keep a ring of particles, have these waves passing through this ring, and stretch and squeeze these particles as the waves travel through this arrangement. Based on this principle, we try to make a detector and it helps us look for the gravitational waves. “The tricky part is to be able to properly isolate the effect of these waves from other disturbances!” he added.
Why this Research?
Dr. Sengupta discussed that gravitational waves are the fundamental predictions of Einstein’s theory of gravity. The strongest components of these entities have been known for the last two years. All of the detections made so far, by his research group, were based on these strongest components known as the quadruple components. He expressed, “But, this time we wanted to explore the other subtler components of these waves, which were only known in theory before our study. People had worked out and estimated what they might look like and what could be their effect on signals from black-holes and so on. Moreover, the LIGO (Laser Interferometer Gravitational-Wave Observatory) detectors are biased towards equal mass black-holes. Hence, our chances to observe these weak parts were very few. We were very lucky to identify a system with asymmetric masses, where one black-hole was about three times heavier than the mass of the other. It, sort of, led to the magnification and enhancement of these fainter components.”
The Workflow
Think of a prism. It scatters white light into seven different (rainbow) colors. Simply speaking, it is helping us to understand the different components of the white light. “Along similar lines, for this research, our team members utilized a technique that was developed long back. It is known as Fourier transform and allows us to observe a stretch of a signal in time. It projects this signal onto sinusoids of different frequencies. Similar to a prism, this transform is able to decompose a particular signal into its constituent frequencies,” he stated.
When a gravitational wave is embedded in the data, the wavelet transformations (similar to Fourier transforms) give an estimate of the amount of energy present in different frequencies, as we move along in time. This process gives us some shapes and we need to identify whether they match with that of the conventional gravitational-wave signals.
Simulation of the binary black hole merger GW190412.
Collaborations Explained
Gravitational-wave detectors are very unique. If we take the case of telescopes, it is possible to get better images of a particular star on increasing the size (along with sophistication, of course!) of this apparatus. But, gravitational waves do not work like that. They work best when we have a network of these kinds of detectors. There are two such detectors in the USA (called LIGO) and one in Italy (VIRGO). Furthermore, there is another detector coming up in India, known as LIGO-India. It is a joint collaboration between the Indian and US scientific authorities. The reason to employ several detectors is that we can effectively localize the source. Detectors can be thought of as giant ‘ears’, but only one of them will not be able to capture the essence of feeble components, and hence, there is a need to triangulate a source.
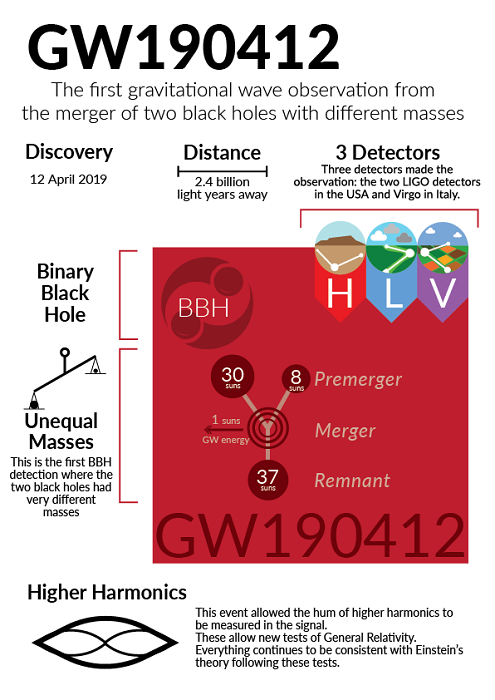
In the words of Dr. Sengupta, “The detectors used by our team were functional 24×7 for several months and recorded these components based on the interactions between the gravitational waves. The same event was observed on all the three detectors but at slightly different time points (of the order of a few milliseconds)! This process is very crucial since it helps in accurately locating the source in the sky as well as in time.”
Another point worth mentioning is that there is a lot of noise in the universe and these signals are very faint. Looking at these events through more than one detector is also helpful in confirming that they actually happened. We can think of it in this way — essentially, noise levels captured by detectors that are geographically apart will not be correlative. But, a particular gravitational wave signal coming from space will be exactly the same for all these detectors. On cross-correlating, the noise will be canceled out and we will be left with our desired result.
Unraveling Some Interesting Mysteries
From the shape of any signal, it is possible to extract almost all of its parameters using certain standard inference techniques and statistics. Suppose, if we are given a signal embedded in noise and we have an idea-model of what the signal might look like, we would be able to gain an understanding of its parameters such as the massivity of a particular system, its distance from us, its movement, and so forth.
Each of these traits has an imprint on the signal. “Therefore, if we can measure the signal very precisely, we can actually go back and construct the identity of that system and various other mysterious aspects,” he described.
In the expanding universe, distance is the same as time. The farther away the system is, we are basically looking back in time. Now, we can utilize all this information to work out when a phenomenon, like a black-hole collision, might have happened so that we are receiving its signals right now. Different parts of cosmology come together from which we infer that, say, a particular collision took place two billion years ago. This interesting area is a very young field in Physics research. By looking at phenomena that would have happened way back in time, we can actually piece together the matter-distribution of the universe. Simply put, we can solve several mysteries of how this universe came into being! Isn’t it interesting?
According to Dr. Anand Sengupta, “We need many more observations to be able to compile enough statistics so that we can completely base our conclusions from gravitational waves alone. Right now, we have about a dozen such observations as of now. But people have already started working on this idea that if one has enough binary black-hole observations coming from different parts and directions of the universe, can they be used to say how these objects are distributed throughout it? Hence, we are basically going in the past to explore the picture of the universe way back in time. It is very exciting and might even be the only way to unveil several breathtaking processes of our very early universe!”
— — — — — — — — — — — — — — — — — — — — — — — — — —— — — — — — — — — — — — — — — — — — — — — — — — — —— — — — —
* This story has also been published on Medium. The video of the same is available below.
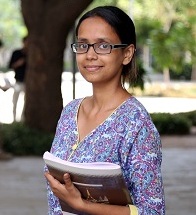
APEKSHA SRIVASTAVA
Senior Project Associate